The submillimetre and near-millimetre spectral region, from 300 μm to 1.2 mm, offers great potential for spectroscopy. Emission from astronomical sources at these wavelengths takes the form of both continuum (ice, dust) and line (atomic, molecular) components. The continuum emission provides information on the nature of the emitting solid particulates and their physical properties; line emission provides information on the column abundance of the atomic and molecular species and their physical conditions. Ground-based observations are possible at these wavelengths because the Earth's atmosphere transmits electromagnetic radiation in spectral bands known as "windows" which occur between the strong absorption lines of water vapour.
In collaboration with a team of colleagues, I have been engaged in a campaign of observations to measure the emission spectra of the planets, as well as galactic and extragalactic sources, in the submillimetre and near-millimetre windows using a Fourier Transform Spectrometer (FTS) at the James Clerk Maxwell Telescope (JCMT).
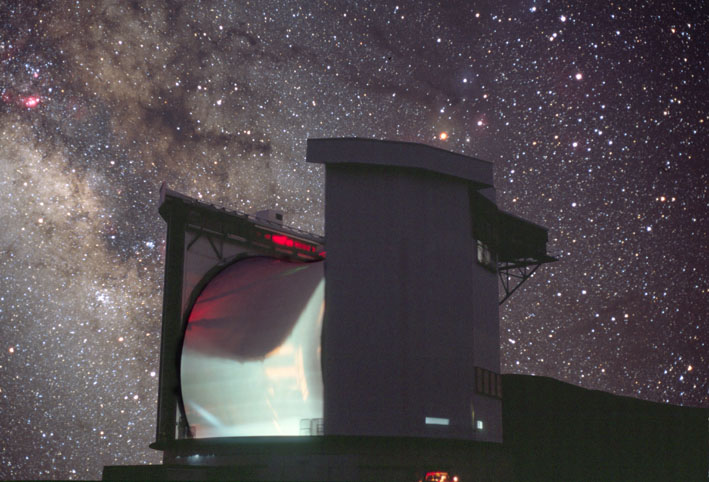
At submillimetre wavelengths, the transmittance of the terrestrial atmosphere is determined primarily by the amount of water vapour in the line of sight. A high-altitude observing site is therefore an absolute requirement. Unfortunately, even though the summit is normally well above most of the weather, the water vapour content of the atmosphere above the telescope can be quite variable.
The telescope has a segmented primary mirror of diameter 15 m and is the largest telescope in the world devoted to submillimetre astronomy. The JCMT is operated by the Joint Astronomy Centre in Hilo, Hawaii on behalf of the parent organizations: the Particle Physics and Astronomy Research Council in the United Kingdom, the National Research Council of Canada and The Netherlands Organization for Scientific Research.
Our group is responsible for developing the imaging spectrometer for the SCUBA-2 instrument at the JCMT.
Please see the SCUBA-2 FTS project page for more information.
A polarizing Fourier Transform Spectrometer (FTS) based on the two beam Martin-Puplett design (IR Phys. 10, 105, (1969)) was developed by our group for use at the JCMT starting in 1991. This instrument was ideally suited to observational programs requiring a broad spectral coverage at intermediate resolution (R ~ 40 km/s at 350 μm). The advantages of the polarizing interferometer include a high and uniform efficiency over a wide wavelength range, access to two input ports and well defined radiation paths within the interferometer, essential in the calibration of resulting spectra. It is fundamentally similar to the Michelson interferometer in that it uses 2-beam interference to generate an interferogram, from which the spectrum can be recovered by a Fourier transform. The maximum optical path difference between the two beams was 1.2m, which provided a spectral resolution of 125 MHz (0.004 cm-1). The FTS coupled to the Nasmyth focus of the telescope and received the f/35 beam.
Early measurements with this spectrometer used the JCMT's facility bolometric detector, UKT14. Analysis of the data obtained with this detector, however, revealed the presence of pervasive channel fringes in the spectra due to parasitic Fabry-Perot effects arising from parallel surfaces in the detector's internal optical system, a feature which is irrelevant for conventional photometry but which greatly complicates the spectral calibration. These fringes can be seen in some of the science results below. In part to circumvent this problem, a dual-polarisation 3He detector system was commissioned on the telescope in August 1996. The detector was photon-noise limited in the 350 μm and 450 μm bands, and nearly so at 850 μm and 1400 μm; the noise equivalent power, excluding "sky noise" caused by atmospheric turbulence, is < 5x10-16 W/sqrt(Hz), which placed this detector amongst the best 0.3 K bolometers in the world.
A new FTS using a Mach-Zehnder design and novel intensity beamsplitters was designed in 1999 and operated on the JCMT as a visiting instrument between 2000 and 2008. The MZ spectrometer was built using the same basic design as the Herschel-SPIRE instrument, and its use both at the JCMT and at the U of L labs enabled the novel SPIRE beamsplitters to be tested. The SPIRE data reduction software written by the group as part of a CSA contract was based on experience gained with this instrument.
The instrument papers for the original design, as well as the new Mach-Zehnder design can be found in the papers section.
Scan Mode |
Rapid scan. Maximum scan time ~ 60 s. |
|||||||||||||||
Spectral Bands
Click here for picture |
|
|||||||||||||||
Resolution |
0.004 cm-1 (125 MHz), R ~ 7.5 x 103 or 40 km/s @ 30 cm-1 |
|||||||||||||||
Beam Width |
~ 6" - 19" (FWHM) |
|||||||||||||||
NET (300 s integration) |
~ 1 K per resolution element (0.004 cm-1 or 125 MHz) 850 and 1100 µm
bands ~ 5 K per resolution element (0.004 cm-1 or 125 MHz) 350 and 450 µm bands |
The sensitivity of the FTS is to a large extent determined by atmospheric conditions, and in particular variations in atmospheric transmission during a scan. Based on results from the May 1998 observing run, under stable conditions NET's of ~ 1 K in the 850 -- 1300 µm bands per resolution element per 300 s integration and ~ 5 K in the 350 -- 450 µm bands per resolution element per 300 s integration can be obtained. For a given S/N, integration times increase when the source does not fill the beam (due to the dilution factor) and decrease with lower spectral resolution (due to the wider spectral bins). The noise levels have been independently confirmed with the JCMT pointing measurements which show continuum NEFD's of .5 Jy Hz-1/2 @ 1100 µm. This value includes the efficiency of the FTS and is a factor ~ 4 better than that measured with UKT14. |
|
![]() |
O3 lines in the atmospheric transmission spectra have been used to determine
the resolution and accuracy of the spectra. The O3 lines provide a critical
test of the performance of the FTS. The lines have a symmetrical shape with widths
(FWHM) ~ 0.005 cm-1. The accuracy of the line positions has been measured to
be 0.0002 cm-1. Furthermore, the FTS is intrinsically stable as evidenced by
a 4 hour integration in the 350 µm band searching for maser emission from MWC349.
The S/N of these spectra increased exactly as the (#spectra)1/2.
|
The objective of this campaign of observations was to measure the emission spectra of the planets in the submillimetre atmospheric windows. For the giant planets, these spectra consist of two components:
- A continuum spectrum is produced in the deep atmosphere, mainly by molecular hydrogen (the dominant atmospheric constituent). Although the hydrogen molecule is symmetric and therefore has no permanent dipole moment, it does emit electromagnetic radiation under the conditions of high pressure and high temperature which exist deep in the giant planet atmospheres.
- An absorption line spectrum is produced against this background by other atmospheric constituents at higher (colder) altitudes. Most of the spectral lines at submillimetre wavelengths are due to rotational molecular transitions. Detailed measurements of the shapes of these spectral features, for which high spectral resolution is required, can be analysed to determine the abundance and distribution of the constituents which cause them.
Each constituent has a different story to tell about the physical and chemical conditions in the atmosphere in question. Here are some of the early results from this programme:
Naylor et al. (1994) reported the first detection of the carbon monoxide J=3-2 absorption feature in Neptune, centred at 354.5 GHz. CO was unexpectedly discovered in the stratosphere of Neptune in 1992 using heterodyne instrumentation, and this is the first detection of a tropospheric absorption component. The measured spectrum is indicated in red and a synthetic spectrum, which was derived for a uniform vertical distribution of carbon monoxide in the neptunian atmosphere, is in yellow. Despite the presence of channel fringes in the data (see above), the agreement between the two is encouraging. Further measurements of this line and of other CO lines are planned with the new detector system, with which we hope to eliminate the channel fringes and determine the tropospheric CO abundance more precisely. |
![]() (Click for large view) |
A 1981 paper claimed to have detected hydrogen cyanide in Jupiter's atmosphere, with a mole fraction in the upper troposphere of 1.8×10-9. This was a surprising discovery since HCN should not be stable at observable levels, and over the next several years various theories were advanced to explain its formation. After several attempts to detect the strong submillimetre absorption lines of HCN, Davis et al. (1997) conclusively demonstrated that HCN does not exist in Jupiter at the abundance level originally claimed. The figure indicates our upper limits for five different assumed vertical distributions. |
![]() (Click for large view) |
In the paper by Davis et al. (1997) , we reported the first-ever measurement of the Jupiter continuum spectrum in the 850µm window. Calibration of the continuum is extremely difficult because of various effects, including for example fluctuations in atmospheric transmittance, which must be taken into account. In the present case, the continuum was obtained by ratioing against the Sun to remove the extended-source channel fringes. The red curves in the figure represent the measured spectrum and the error envelope; the error here is almost entirely due to the uncertainty in the solar temperature at these wavelengths. The blue and orange curves represent two synthetic spectra, one (blue) for a nominal ammonia abundance and one (orange) for an enhanced ammonia abundance; the latter is a better fit to our measurements. The hatched region represents a photometric measurement of the jovian brightness temperature by Griffin et al. (1986), with which our own measurement is in excellent agreement. |
![]() (Click for large view) |